Oxidative‐inflammatory damage in cirrhosis:
Effect of vitamin E and a fermented papaya preparation

Abstract
Background and Aim: Oxidative DNA damage occurs as an early event in hepatitis C virus (HCV) infection and is an indication of the potential for carcinogenesis. The aim of this study was to test a novel antioxidant/immunomodulator in patients with HCV‐related cirrhosis.
Methods: The study group consisted of 50 patients with HCV‐related cirrhosis with transaminase values less than twofold increased (alanine aminotransferase [ALT] < 80 IU/L). Patients underwent a standardized food‐vitamin composition assessment and were assessed for dietary intake, nutritional status and iron level. Patients were randomly allocated into two groups and then given either α‐tocopherol 900 IU/day or 9 g/day of a fermented papaya preparation (FPP, Immun‐Age, Osato Research Institute, Gifu, Japan) at bedtime for 6 months. Ten healthy subjects served as controls. Patients were checked monthly for: routine tests, redox status (reduced glutathione, glutathione peroxidase, oxidized glutathione, malondialdehyde), plasma α‐tocopherol, 8‐hydroxy‐deoxy‐guanidine (8‐OHdG) level in circulating leukocyte DNA and serum levels of cytokines.
Results: Patients with cirrhosis showed a significant imbalance of redox status (low antioxidants/high oxidative stress markers) (P < 0.005 vs controls). Neither treatment regimen affected transaminases as a whole. However, vitamin E supplementation almost normalized ALT only in the limited vitamin‐E‐deficient subgroup. A significant improvement of redox status was obtained by both regimens. However, only FPP significantly decreased 8‐OHdG and the improvement of cytokine balance with FPP was significantly better than with vitamin E treatment (P < 0.05).
Conclusions: Although the present data seem to suggest a potential supportive role of antioxidants/immunomodulators as FPP in HCV patients, more studies are needed to substantiate their effect on the natural history of the disease.
Introduction
The liver is one of the most susceptible organs to oxidative‐related cellular damage and DNA mutagenesis, and oxidative stress has been implicated as a causative factor in alcoholic and non‐alcoholic liver disease. In alcoholism there is an understandable link with ethanol metabolism due to the production of reactive oxygen species (ROS) such as superoxide and hydroxyl radicals. For non‐alcoholic liver disease, a complex interplay between malnutrition, trace elements abnormalities, glutathione depletion and several virus‐related cellular injuries are indicated.1 A key factor causing oxidative DNA damage is formation of hydroxyl radicals, which can alter purine and pyrimidine bases and react with deoxyribose damaging the phosphodiester DNA structure. Oxidative modification of pyrimidine and/or purine bases occurs through addition of hydroxyl radicals to the π bonds of the bases, to C5 and C6 of pyrimidines and to C4 and C8 of purines.
Stable oxidative damage products such as 8‐hydroxy‐deoxy‐guanidine (8‐OHdG) are molecular markers of pathology.2 Oxidative DNA damage, namely 8‐OHdG generation, has been indicated as an early event in hepatitis C virus (HCV) infection and as a marker of liver damage. Persistent genomic changes are factors giving rise to carcinogenesis, as has also been suggested in patients undergoing chronic hemodialysis.3 Therapeutic interventions with antioxidants aiming to curb 8‐OHdG as a means to prevent hepatocarcinogenesis have recently been confirmed in an experimental liver cancer model where an herbal preparation, sho‐saiko‐to, appeared to be effective.4 This preparation is not amenable to widespread use given the severe side‐effects reported in clinical practice.5-7 The oretically, however, a nutraceutical approach effective in maintaining redox balance and an immunomodulator without adverse effects would be desirable.
Experimental studies using a non‐genetically modified antioxidant/immuno‐stimulating and nitric oxide‐modulating fermented papaya preparation (FPP) have been described.8-10 FPP was found to posses highly protective antioxidant properties despite lacking any specific antioxidant vitamin11-14 (Table 1). Such studies have been followed by clinical investigations.15-19 In particular, recent gastroenterology studies19 have demonstrated that FPP was able to significantly decrease the oxidative stress in gastric mucosa affected by longstanding chronic atrophic gastritis associated with metaplasia and importantly to curb the mucosal concentration of 8‐OHdG. Moreover, it has been shown in patients with HCV‐related chronic liver disease that high tumor necrosis factor (TNF)‐α levels are associated with the degree and progression of inflammation20 while the concentration of the soluble TNF p75 receptor seems to be linked to mortality.21 The aim of the present investigation was to test supplementation with vitamin E or FPP in a group of patients with established HCV‐related liver cirrhosis.
Composition | Amount (per 100 g) |
---|---|
General | |
Carbohydrates | 90.7 g |
Moist | 8.9 g |
Proteins | 0.3 g |
Fats | Absent |
Ashes | 0.1 g |
Fiber | Absent |
Vitamin B6 | 17 µg |
Pholic acid | 2 µg |
Niacin | 240 µg |
Iron | 0.29 mg |
Calcium | 2.5 mg |
Potassium | 16.9 mg |
Magnesium | 4.6 mg |
Copper | 14 µg |
Zinc | 75 µg |
Amino acids | |
Arginine | 16 mg |
Lysine | 6 mg |
Hystidine | 5 mg |
Phenylalanine | 11 mg |
Tyrosine | 9 mg |
Leucine | 18 mg |
Isoleucine | 9 mg |
Methionine | 5 mg |
Valine | 13 mg |
Alanine | 12 mg |
Glycine | 11 mg |
Proline | 8 mg |
Gluthamic acid | 37 mg |
Serine | 11 mg |
Treonine | 8 mg |
Aspartic acid | 27 mg |
Triptophane | 2 mg |
Methods
The study group consisted of 50 patients (29 male, 21 female), mean age 62 years (range 54–75 years), with Child A–C, genotype 1 HCV‐related cirrhosis without a history of alcohol consumption for the past 10 years. All patients had alanine aminotransferase (ALT) levels that were abnormal but less than 80 IU/L. Patients were carefully interviewed with special attention to their dietary vitamin and iron intake using a standardized food‐composition table.22
Exclusion criteria were: hemocromatosis, Wilson's disease, alpha‐1‐antitrypsin deficiency, autoimmune diseases, alcohol consumption, hepatocellular carcinoma, recent variceal hemorrhage or scheduled endoscopic session of variceal banding, any other malignancy, chronic illness requiring steroids, immunosuppressive agents, allopurinol treatment, antiviral or non‐steroidal anti‐inflammatory drugs, concomitant use of vitamin/food supplements, strenuous physical exercise, chronic renal failure, arterial hypertension, overt cardio‐respiratory abnormality and high consumption of caffeine‐containing food or beverages.
Patients were randomly allocated into two groups (25 patients each), previously matched for dietary intake, serum iron concentration and iron dietary intake (median: 8.6 mg/day, range: 6.9–10.4 mg/day) and body mass index. Each group received a supplement at bedtime for 6 months. Group A received α‐tocopherol 900 IU/day and group B received FPP 9 g/day (Immun‐Age, made under ISO 9001 [production quality] and ISO 14001 [environmental protection] regulations from biofermentation of carica papaya, pennisetum purpureum and sechium edule; Osato Research Institute, Gifu, Japan).
Ten healthy subjects who were not taking any food or vitamin supplements were matched for age and sex with the patient population and were used as the control group.
After an overnight fast, venous blood samples were taken. A dry tube for serum, a citrate‐containing tube and an ethylenediaminetetra‐acetate (EDTA)‐containing tube for plasma were used for clinical chemistry analyses. On the examination day, blood samples were taken for routine testing by automated standardized procedures (Hitachi 911 using commercial kits, Hitachi Medical Corporation, Tokyo, Japan) and for further studies as described below.
Assessment of redox status
For assessing the variation of the blood redox antioxidant status, plasma was prepared from heparinized whole blood (10 mL) by centrifugation at 2000 g for 15 min at 4°C. The following parameters were measured: reduced glutathione, glutathione peroxidase and oxidized glutathione as described23 using hemoglobin catalyzed oxidation of 10‐N‐methylcarmoyl‐3,7‐dimethylamino‐10‐H‐phenothiazione after treatment with phospholipase D. Accordingly, cumene hydroperoxide was used as a standard.
Plasma content of α‐tocopherol was measured by high performance liquid chromatography (HPLC). Briefly (1 mL) aliquots of plasma were mixed with 1 mL of 100 mmol/L sodium dodecyl phosphate solution in water, 2 mL of absolute ethanol and 1 mL of n‐heptane and shaken for 1 min. After 15 min extraction in the dark, the heptane phase was separated by centrifugation, and 50 µL aliquots were used for HPLC assay. Values were read by a fluorescence detector set at 296 nm excitation and 325 nm emission.
Determination of plasma malondialdehyde
Malondialdehyde (MDA) was measured in frozen, EDTA‐containing plasma samples after reaction with thiobarbituric acid (TBA) using a slight modification of the HPLC method described by Rabl et al.24 Before assay, plasma samples were thawed and 100 mL aliquot was immediately mixed with 100 mL water, 300 mL of phosphoric acid 0.15 mol/L, 10 mL butylated hydroxytoluene [BHT] 0.2% methanolic solution, and 100 mL 0.6% TBA, and then incubated for 60 min at 95°C. The chromogen was extracted with 1.25 mL butanol‐1 and analyzed by HPLC with fluorometric detection (emission wavelength: 550 nm; excitation wavelength: 525 nm). The MDA‐TBA adduct was calibrated with tetramethoxypropane standard solutions and processed like the plasma samples.
Analysis of 8‐OHdG in circulating leukocyte DNA
Leukocytes were isolated after centrifugation over 100 µL Histopaque 1077 at 200 g for 3 min at 4°C, washed once in phosphate‐buffered saline (PBS; pH 7.4) and centrifuged again. Then the leukocyte layer was collected and tested for 8‐OHdG by HPLC as follows. DNA was isolated and purified from leukocytes as described25 with minor modifications. Briefly, after homogenization in 1 mL of ice‐cold buffer (0.1 mol/L NaCl, 10 mmol/L EDTA, 10 mmol/L 2‐mercaptoethanol, and 0.5% Triton X‐100, pH 8.0) and centrifugation for 10 min at 1000 g at 4°C. The samples were then resuspended in 7.5 mL of lysis solution (120 mmol/L NaCl, 10 mmol/L Tris, 1 mmol/L EDTA, and 0.5% SDS, pH 8.0) with 20% BHT. RNA and protein were digested by incubation with RNase or proteinase K for 30 or 60 min at 55°C, respectively.
After extraction, DNA was precipitated by the addition of 5 volumes of ethanol (−20°C). The isolated DNA (100–400 µg) was dissolved in 200 µL of 20 mmol/L sodium acetate (pH 5.0), denatured by heating for 5–10 min at 95°C, and cooled on ice. The DNA samples were digested to nucleotides by incubating with 12 units of nuclease P1 for 30 min at 37°C. Next, after adding 20 µL of 1 mol/L Tris‐HCl (pH 8.0) and 4 units of alkaline phosphatase, the samples were incubated for 1 h at 37°C. The resulting deoxynucleoside mixture was filtered through a Millipore filter (0.22 µm) and analyzed using a HPLC‐electrochemical detection (ECD) system equipped with a CoulArray 5600 EC detector (ESA Laboratories, Inc., Chelmsford, MA, USA).
The amount of 8‐OHdG was compared with the quantity of deoxyguanosine detected in the same sample by UV absorbance at 260 nm. 8‐OHdG and 2′‐deoxyguanosine were measured using different channels and oxidation potentials of 300 and 900 mV, respectively. The results are expressed as the ratio of the absorbance peak of 8‐oxo‐deoxyguanosine adducts to that of 2′‐deoxyguanosine adducts ×105.
Circulating levels of cytokines and cytokine receptors
Serum was collected after centrifugation at 4°C and samples were stored in aliquots at −20°C until use. Levels of TNF‐α and sTNF‐Rp75 were measured using commercial sandwich‐type ELISA kits following the procedure recommended by the manufacturers (Biosource Technologies, Nivelles, Belgium and R & D Systems, Minneapolis, MN, USA).
Briefly, two diluted serum samples were added to plates coated with antibody and incubated for 2 h at 37°C. Afterwards, each well was washed five times with washing buffer, then peroxidase‐labeled secondary antibody was added to each well and the plate was incubated for 1 h at 37°C. Then each well was washed in a similar manner and the plate was incubated with tetramethylbenzine for 20 min at room temperature. The reaction was stopped by adding 1 N sulfuric acid. Absorbance was measured at 450 nm using a spectrophotometric analyzer. Sample concentration was derived from a standard curve. The following sensitivity limits were achieved in standard curves: TNF‐α, 19.86 pg/mL; sTNF‐Rp75, 8.5 pg/mL.
Statistical analysis
Data are expressed as mean ± SD. Statistical analysis was performed with the non‐parametric Mann–Whitney U‐test using the statistics software package. Statistical significance was set at P < 0.05. Spearman's correlation analysis was used to examine the correlations between data sets.
Results
Two patients (one from each group) were removed from the study because one contracted the flu with a supervening acute bronchitis requiring paracetamol and antibiotic prescription and the other missed FPP supplementation for 1 week while on holiday and being in good health condition. Altogether, 48 fully complying patients completed the observational study.
No significant weight change was observed. As a whole, routine blood tests were not affected by any of the supplement treatments (data not shown). The oxidized glutathione serum level in patients with cirrhosis was comparable to healthy subjects (Table 2) and remained unchanged by supplementation. However, as compared to healthy controls, reduced glutathione and glutathione peroxidase were significantly lower in cirrhotic patients (P < 0.05) and were comparably improved by either FPP or vitamin E regimens (P < 0.05). In patients with cirrhosis, serum MDA levels were significantly higher (P < 0.01 vs healthy control) and supplementation brought about a comparable partial improvement (P < 0.05 vs baseline values).
Parameter | Control | Vitamin E supplement | FPP supplement | ||
---|---|---|---|---|---|
Baseline | 6 months | Baseline | 6 months | ||
GSH (µmol/L) | 972 ± 52 | 561 ± 34 | 786 ± 69* | 521 ± 54 | 713 ± 72* |
GSSG (µmol/L) | 29 ± 3 | 26 ± 4 | 27 ± 5* | 29 ± 4 | 27 ± 8* |
GSH/GSSG ratio | 33.5 | 21.6 | 29.1* | 18.0 | 26.4* |
MDA (µmol/L) | 1.1 ± 0.3 | 3.2 ± 0.6 | 1.7 ± 0.2* | 3.4 ± 0.5 | 1.9 ± 0.2* |
α‐tocopherol (µmol/L) | 29.2 ± 1.2 | 14.4 ± 0.7 | 23.8 ± 0.3* | 15.6 ± 06 | 15.9 ± 0.8* |
- * P < 0.05 vs vitamin E supplement group. Values shown as mean ± SD. FPP, fermented papaya preparation; GSH, reduced glutathione; GSSG, oxidized glutathione; HCV, hepatitis C virus; MDA, malondialdehyde.
Four patients (16.6%) in the α‐tocopherol group and five patients (20.8%) in the FPP group showed a deficiency of vitamin E level (serum levels below 12.5 µmol/L as a 5% percentile of healthy controls). This was returned to within normal limits by α‐tocopherol supplementation but not by FPP supplementation (data not shown). Three of the four vitamin E‐deficient patients who were supplemented with α‐tocopherol totally normalized their transaminase levels (data not shown).
As compared to controls, patients with liver cirrhosis showed significantly higher accumulation of 8‐OHdG in circulating leukocytes (P < 0.01, Fig. 1). This impairment remained unchanged by α‐tocopherol supplementation; however, it was partially and significantly improved in the FPP‐supplemented group (P < 0.05).
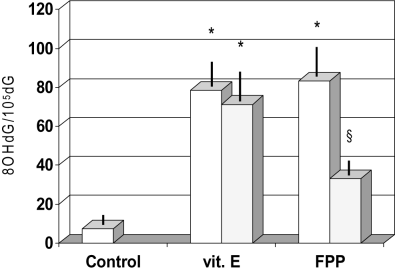
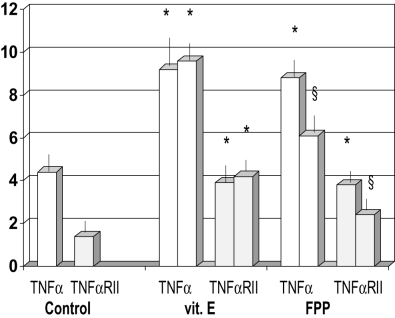
Figure 1
Effect of fermented papaya preparation (FPP) on concentration of 8‐OHdG in circulating leukocytes. *P < 0.001 vs control; §P < 0.05 vs vitamin E group and control.
Leukocyte DNA damage showed a correlation only with the age of patients, as an independent variable (Table 3). Furthermore, patients with liver cirrhosis showed an elevated serum level of TNF‐α and of its soluble p75 receptor (P < 0.001 vs healthy controls, Fig. 2), the latter being significantly related to Child–Pugh score (r: +0.67, P < 0.05, data not shown) among the spectrum of biochemical and clinical parameters tested. Although vitamin E supplementation did not affect this abnormality, FPP supplementation significantly lowered their values (P < 0.05).
Parameter | 8‐OHdG in circulating leukocytes |
---|---|
GSH | −0.19 |
GSH‐Px (ng/mL) | −0.12 |
GSSG (µmol/L) | −0.07 |
MDA | +0.31 |
α‐Tocopherol (µmol/L) | +0.27 |
BMI (kg/m2) | −0.14 |
Iron | +0.19 |
Age (years) | +0.46* |
Child–Pugh score | +0.23 |
Duration of disease (years) | +0.07 |
- * P < 0.05. Values shown as r (correlation coefficient). BMI, body mass index; GSH, reduced glutathione; GSH‐Px, glutathione peroxidase; GSSG, oxidized glutathione; MDA, malondialdehyde.
Figure 2
Effect of fermented papaya preparation (FPP) on concentration of tumor necrosis factor (TNF)‐α and TNF‐α receptor type II (RII). *P < 0.001 vscontrol; §P < 0.05 vs vitamin E
group and control.Discussion
In the course of liver disease, chronic inflammatory events26, 27 and oxidative stress28-30can lead to DNA damage. Indeed, hepatocellular carcinoma frequently develops in patients with chronic hepatitis and liver cirrhosis; it is considered to be a part of the natural history and an unavoidable event occurring at a rate of 1 per 1000 cases. Oxidative damage of nuclear DNA is linked to a number of cancerous transformations. Matsumoto et al.31recently reported that in the non‐cancerous region of HCC patients, the concentration and labeling index of 8‐OHdG was higher in recurrent than in non‐recurrent cases. The presence of 8‐OHdG in DNA leads to GC to TA transversion conditions, which can trigger a mutagenetic process and many observations indicate a direct correlation between in vivo 8‐OHdG accumulation and carcinogenesis.32 Hence measuring 8‐OHdG may be a useful biomarker for detecting liver injury of environmental origin33 and in nonalcoholic fatty liver disease.34 However, it has been shown that impaired redox status even in symptom‐free HCV may represent a negative prognostic factor.35 Whatever the regimen employed, antioxidant supplementation significantly improved the oxidant/antioxidant balance observed as a partial restoration of glutathione status and decreased lipid peroxidation with vitamin E or FPP, which exhibits antioxidant activity (levels of MDA).
There are several reports indicating that glutathione synthesis might be impaired in patients with nonalcoholic liver cirrhosis.36, 37 In our study, MDA did not achieve a significant correlation with leukocyte 8‐OHdG concentration. However, an abnormally high MDA level has been linked with chromosomal breakage factors, which has a potential carcinogenetic role.38 Interestingly, unlike vitamin E, FPP significantly reduced leukocyte 8‐OHdG concentration. Farinati et al.39 found a significant correlation between 8‐OHdG content of circulating leukocytes and levels in liver tissue, and the same research group has also suggested that the 8‐OHdG level in leukocytes is a reliable marker of the severity of liver disease.1
The high reactivity and short half‐life (∼10−9 s) of hydroxyl radicals implies close proximity to DNA bases and this might help explain why vitamin E in the present study failed to protect DNA as well as its reported lack of correlation with leukocyte DNA damage.40-42 At the end of the study, FPP supplementation remarkably decreased leukocyte 8‐OHdG concentration by over 40%. This effect was much greater than the 21% decrease of urinary 8‐OHdG excretion observed in subjects after quitting smoking, and which did not show further improvement at the end of the 26‐week period of smoking cessation.43
It has been demonstrated in the course of HCV‐related liver disease that hepatocellular damage may be triggered by a number of immunological reactions occurring at the cell surface.44 TNF in particular elicits further oxidative damage. The actions of TNF‐α are mediated by two separate TNF receptors, receptors 1 (p55; TNFR1) and 2 (p75; TNFR2).45Quite recently there have been several reports suggesting a role of soluble TNF receptors in HCV‐related liver diseases paralleling its severity and histological activity.46-48 In particular, the concentration of the soluble p75 receptor correlates with disease progression and mortality.21, 49 In our study, despite only a marginal hypertransaminasemia, TNF‐α and TNFR2 receptor were significantly elevated. Both antioxidant regimens comparably decreased TNF‐α levels while TNFR2 was significantly lowered only with FPP supplementation.
Interestingly, Kakumu et al.47 reported that interferon treatment significantly decreased the level of circulating interleukin (IL)‐10 and IL‐15 but not of soluble TNF receptors. However, our population was composed of patients with cirrhosis with mild hypertransaminasemia and whose baseline level of TNFR2 was comparable to the group of asymptomatic HCV carriers in the study of Kakumu et al.48 Moreover, we found that, although to a lesser degree as compared to Look et al.,50 18% of our patients were vitamin E deficient. Interestingly, 60% of the vitamin E‐deficient patients who were also supplemented with vitamin E 900 IU totally normalized their transaminase level.
Another interfering factor might also be iron status and iron is likely to be involved in the HCV infection‐hepatocarcinogenesis transformation. This was the rationale for choosing to match the treatment groups for iron status. Indeed, it is likely that inflammatory‐related cytokines, including TNF‐α, induced by hepatic inflammation would stimulate iron uptake via up‐regulation of transferrin receptor expression in hepatocytes.51 In this regard, the cytokine‐mitigating properties of FPP might be of potential benefit in the clinical setting.
Despite the therapeutic armamentarium being enriched by new effective antiviral drugs and regimens in recent years, there are a substantial percentage of non‐responders whose cirrhotic transformation cannot be prevented. Moreover, patients with established HCV‐related cirrhosis are often not eligible for antiviral treatment. Although the ultimate therapeutic target is to eradicate HCV, antioxidant therapy might offer a worthwhile adjunctive tool, especially in long‐term management of patients when several, yet to be fully elaborated, metabolic‐nutritional abnormalities occur.52 Indeed, it has been suggested that the generation of ROS even at such low levels that are unable to bring about overt parenchymal cell death, when chronically occurring for a long time, can lead to accumulation of 8‐OHdG in DNA53 and such genomic abnormalities have been described even at a stage of chronic hepatitis.39, 54,55 However, such complementary support still deserves further studies and has to be carefully formulated to avoid any uncontrolled vitamin supplementation. This holds particularly relevant when considering the albeit controversial meta‐analysis questioning the benefit of high‐dosage of vitamin E.56